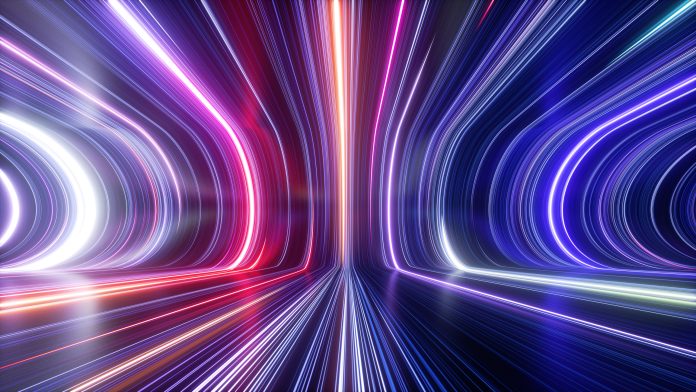
MIT engineers have achieved a major leap towards fault-tolerant quantum computing by demonstrating an order-of-magnitude stronger nonlinear light-matter coupling. This breakthrough promises nanosecond-speed quantum information readout and processing, significantly accelerating the path to practical quantum computation
In a significant stride towards realising the full potential of quantum computers, researchers at MIT have demonstrated an unprecedentedly strong nonlinear coupling between artificial atoms and photons.
This crucial advancement, published in Nature Communications, could enable quantum information readout and processing in mere nanoseconds, dramatically improving the speed and accuracy necessary for fault-tolerant quantum computation, a key hurdle in the field.
Overcoming bottlenecks in quantum processing
Quantum computers hold the promise of revolutionising fields ranging from materials science to machine learning. However, their practical application hinges on their ability to perform operations with extreme speed and minimal errors. The readout process, where quantum information is measured, is particularly critical and relies on the strength of the interaction between photons (light particles carrying quantum information) and artificial atoms (qubits, the fundamental units of quantum computers). Errors accumulate rapidly in these delicate systems, necessitating fast measurements and corrections.
The MIT team, led by Yufeng “Bright” Ye, a recent PhD graduate, and senior author Professor Kevin O’Brien, has achieved a nonlinear light-matter coupling approximately ten times stronger than previous demonstrations. This breakthrough, utilising a novel superconducting circuit architecture, could potentially accelerate quantum processors by a similar factor, bringing the era of practical quantum computing closer to reality.
Introducing the quarton coupler: A key innovation
The foundation of this achievement lies in the development of a new type of quantum coupler, dubbed the “quarton coupler,” invented by Ye during his PhD research. This specialised superconducting circuit exhibits the potential to generate exceptionally strong nonlinear coupling, a vital ingredient for executing most quantum algorithms. The coupler’s nonlinearity intensifies with increased current, allowing for more complex and powerful quantum interactions.
“Most of the useful interactions in quantum computing come from nonlinear coupling of light and matter,” explains Ye. “If you can get a more versatile range of different types of coupling, and increase the coupling strength, then you can essentially increase the processing speed of the quantum computer.”
Faster readout for enhanced accuracy
Quantum readout typically involves shining microwave light onto a qubit and detecting a frequency shift in its associated readout resonator, which reveals the qubit’s state (0 or 1). The strength of the nonlinear light-matter coupling between the qubit and the resonator dictates the efficiency of this measurement. The MIT researchers’ design, featuring a quarton coupler connected to two superconducting qubits on a chip, significantly enhances this coupling. By effectively turning one qubit into a resonator and using the other to store quantum information transmitted via microwave photons, they demonstrated the potential for ultrafast readout.
Professor O’Brien emphasises that while this is a fundamental physics demonstration, ongoing work within their group focuses on integrating additional electronic components to create a complete, high-speed readout circuit suitable for larger quantum systems.
Future directions: Matter-matter coupling and fault tolerance
Beyond faster readout, the researchers also demonstrated exceptionally strong matter-matter coupling, another crucial type of qubit interaction for performing quantum operations. This will be a key area of future exploration. The ability to perform both readout and operations rapidly is paramount for fault-tolerant quantum computing. Qubits have a limited lifespan, known as coherence time, and stronger nonlinear coupling allows for more operations and error correction cycles within this timeframe, ultimately leading to more accurate and reliable quantum computations.
“The more runs of error correction you can get in, the lower the error will be in the results,” Ye states. This advancement brings the long-term goal of practical, large-scale quantum computation within closer reach.